Plan Your CRISPR Experiment
Get Started
CRISPR is a powerful system that enables researchers to manipulate the genome like never before. This section will provide a general framework to get you started using CRISPR in your research. Although we will use the example of CRISPR/Cas9 in mammalian cells, many of these principles apply to using CRISPR in other organisms. First, consider the genetic manipulation that is necessary to model your specific disease or process of interest. Do you want to:
- Generate complete and permanent loss of gene expression or function (knockout)?
- Generate a specific mutant allele of a gene (point mutant)?
- Increase or decrease expression of a target gene?
Once you have a clear understanding of your experimental goal, you are ready to start navigating the different reagents that are available for your particular experiment.
Select Your Desired Genetic Manipulation
Different genetic manipulations require different CRISPR components. Selecting a specific genetic manipulation can be a good way to narrow down which reagents are appropriate for a given experiment. Make sure to check whether reagents are available to carry out your experiment in your particular model organism. There may not be a perfect plasmid for your specific application, and in such cases, it may be necessary to customize an existing reagent to suit your needs.
Genetic Manipulation | Application | Cas9 | gRNA | Additional Considerations |
---|---|---|---|---|
Knockout | Permanently disrupt gene function in a particular cell type or organism without a specific preferred mutation | Cas9 (or Cas9 nickase) | Single (or dual) gRNA targeting 5′ exon or essential protein domains | High-fidelity Cas enzymes increase specificity. Dual-nickase approach increases specificity but is less efficient. Each putative knockout allele must be experimentally verified. |
Edit | Generate a specific user-defined sequence change in a particular gene, such as generating a point mutation or inserting a tag | Cas9 (or Cas9 nickase); Base editor | Single (or dual) gRNA targeting the region where the edit should be made | HDR requires a repair template and displays reduced efficiency compared to NHEJ knockout. Base editors can make a limited set of mutations. |
Repress or Interfere (CRISPRi) | Reduce expression of a particular gene(s) without permanently modifying the genome | dCas9-repressor (such as dCas9-KRAB) or dCas9 | gRNA(s) targeting promoter elements of target gene | dCas9-KRAB is more effective than dCas9 alone for mammalian cell lines. |
Activate (CRISPRa) | Increase expression of an endogenous gene(s) without permanently modifying the genome | dCas9-activator (such as dCas9-VP64) | gRNA(s) targeting promoter elements of target gene | Many different activators exist, including the multi-plasmid SAM system. |
Select Expression System
To use CRISPR, you will need both Cas9 and a gRNA expressed in your target cells. For easy-to-transfect cell types (e.g. HEK293 cells), transfection with standard transfection reagents may be sufficient to express the CRISPR machinery. For more difficult cells (e.g. primary cells), viral delivery of CRISPR reagents may be more appropriate. In cases where off-target editing is a major concern, Cas9-gRNA ribonucleoprotein (RNP) complexes are advantageous due to the transient Cas9 expression.
The table below summarizes the major expression systems and variables for using CRISPR in mammalian cells. Some of the variables include:
- Species of Cas enzyme and gRNA
- Species of promoter and expression pattern of promoter for Cas enzyme and gRNA
- Presence of a selectable marker (drug or fluorophore)
- Delivery method
Expression System | Components of System | Application |
---|---|---|
Mammalian expression vector |
|
Transient or stable expression of Cas9 and/or gRNA in a mammalian cell line that can be transfected at high efficiency |
Lentiviral transduction |
|
|
AAV transduction |
|
|
RNA delivery of Cas9 and gRNA | In vitro transcription reactions generate mature Cas9 mRNA and gRNA, which are then delivered to target cells through microinjection or electroporation |
|
Cas9-gRNA ribonucleoprotein (RNP) complexes | Purified Cas9 protein and in vitro transcribed gRNA are combined to form a Cas9-gRNA complex, which is delivered to cells using cationic lipids |
|
Additional Resources:
- CRISPR protocols from Addgene depositors
- JOVE Video Reprint: Protocol for genomic deletions in mammalian cell lines
Select Your Target Sequence and Design Your gRNA
Once you have selected your CRISPR components and method of delivery, you are ready to select a target sequence and design your gRNA.
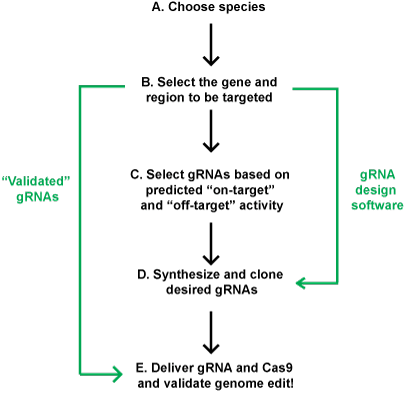
-
Know your cell line/organism and genomic sequence
When possible, you should sequence the region you are planning to modify prior to designing your gRNA, as sequence variation between your gRNA targeting sequence and target DNA may result in reduced cleavage. The number of alleles for each gene may vary depending on the specific cell line or organism, which may affect the observed efficiency of CRISPR knockout or knockin. -
Select gene and genetic element to be manipulated
In order to manipulate a given gene using CRISPR, you will have to identify the genomic sequence for the gene you are trying to target. However, the exact region of the gene you target will depend on your specific application. For example:- To activate or repress a target gene using dCas9-activators or dCas9-repressors, gRNAs should be targeted to the promoter driving expression of your gene of interest.
- For genetic knockouts, gRNAs commonly target 5′ constitutively expressed exons, which reduces the chances that the targeted region is removed from the mRNA due to alternative splicing. Exons near the N-terminus are targeted since frameshift mutations here increase the likelihood that a nonfunctional protein product is produced.
- Alternatively, gRNAs can be designed to target exons that code for known essential protein domains. The benefit of this approach is that even non-frameshift alleles may alter protein function when they occur in important protein domains.
- For gene editing experiments using HDR, it is essential that the target sequence be very close to the location of the desired edit, ideally less than 10 bp away. In this case, it is necessary to identify the exact location where the edit should occur and select a target sequence nearby.
-
Select gRNAs based on predicted on-target and off-target activity
A PAM sequence is absolutely necessary for Cas9 to bind target DNA. As such, one can start by identifying all PAM sequences within the genetic region to be targeted. If there are no PAM sequences for your chosen enzyme within your desired sequence, you may want to consider alternative Cas enzymes (see Cas9 variants and PAM sequences). Once possible PAM sequences and putative target sites have been identified, it is time to choose which site is likely to result in the most efficient on-target cleavage.The gRNA target sequence needs to match the target locus, but it is also critical to ensure that the gRNA target sequence does NOT match additional sites within the genome. In a perfect world, your gRNA target sequence would have perfect homology to your target with no homology elsewhere in the genome. Realistically, a given gRNA target sequence will have partial homology to additional sites throughout the genome. These sites are called off-targets and should be examined during gRNA design. In general, off-target sites are not cleaved as efficiently when mismatches occur near the PAM sequence, so gRNAs with no homology or those with mismatches close to the PAM sequence will have the highest specificity. To increase specificity, you can also consider using a high-fidelity Cas enzyme.
In addition to off-target activity, it is also important to consider factors that maximize cleavage of the desired target sequence or on-target activity. Two gRNA targeting sequences with 100% homology to their DNA targets may not result in equivalent cleavage efficiency. In fact, cleavage efficiency may increase or decrease depending upon the specific nucleotides within the selected target sequence. For example, gRNA targeting sequences containing a G nucleotide at position 20 (1 bp upstream of the PAM) may be more efficacious than gRNAs containing a C nucleotide at the same position in spite of being a perfect match for the target sequence.
Many gRNA design programs can locate potential PAM and target sequences and rank the associated gRNAs based on their predicted on-target and off-target activity (see gRNA design software). Additionally, many plasmids containing validated gRNAs are now available through Addgene. These plasmids contain gRNAs that have been used successfully in genome engineering experiments. Using validated gRNAs can save your lab valuable time and resources when carrying out CRISPR experiments. Read more about how to design your gRNA.
Browse Plasmids: gRNAs
-
Synthesize and clone desired gRNAs
Once you have selected your target sequences it is time to design your gRNA oligos and clone these oligos into your desired vector. In many cases, targeting oligos are synthesized, annealed, and inserted into plasmids containing the gRNA scaffold using standard restriction-ligation cloning. However, the exact cloning strategy will depend on the gRNA vector you have chosen, so it is best to review the protocol associated with the specific plasmid in question (see CRISPR protocols from Addgene depositors). -
Deliver Cas9 and gRNA
Choose a delivery method that is compatible with your experimental system. CRISPR efficiency will vary based on the method of delivery and the cell type. Before proceeding with your experiment, it may be necessary to optimize your delivery conditions. Learn more about CRISPR delivery in mammalian systems. -
Validate genetic modification
Once you have successfully delivered the gRNA and Cas enzyme to your target cells, it is time to validate your genome edit. CRISPR editing produces several possible genotypes within the resulting cell population. Some cells may be wild type due to either (1) a lack of gRNA and/or Cas9 expression or (2) a lack of efficient target cleavage in cells expressing both Cas9 and gRNA.Edited cells may be homozygous or heterozygous for edits at your target locus. Furthermore, in cells containing two mutated alleles, each mutated allele may be different owing to the error-prone nature of NHEJ. In HDR gene editing experiments, most mutated alleles will not contain the desired edit, as a large percentage of DSBs are still repaired by NHEJ.
How do you determine that your desired edit has occurred? The exact method necessary to validate your edit will depend upon your specific application. However, there are several common ways to verify that your cells contain your desired edit, including but not limited to:
- Mismatch-cleavage assay (for NHEJ repaired DSBs): Provides a semi-quantitative readout of the percentage of alleles that have been mutated within a mixed cell population. The region of interest is PCR amplified, PCR products are denatured-renatured, treated with a nuclease that cleaves DNA heteroduplexes, and run on an agarose gel to identify DNA fragments.
- PCR and restriction digest (for HDR repaired DSBs): For small nucleotide edits that introduce a novel restriction site. The region of interest is PCR amplified, digested with the appropriate restriction enzyme and run on an agarose gel to identify DNA fragments.
- PCR amplification and gel electrophoresis (for HDR or NHEJ): For large deletions or insertions, the region of interest can be PCR amplified using primers that (A) flank the region of interest (deletions or small insertions) or (B) span the genome-insert boundary (insertions only). The PCR product is then run on an agarose gel to determine whether the edit was successful.
- PCR amplification, subcloning and Sanger sequencing (for HDR or NHEJ): Provides semi-quantitative assessment of targeting frequency and exact sequence of targeted alleles. Involves PCR amplification of targeted region from DNA, subcloning into a plasmid, and screening individual clones.
- PCR amplification and next-generation sequencing (for HDR or NHEJ): Provides quantitative assessment of the genome edits in your target sequence and can also be used to examine off-target effects.
More information on each of these techniques can be found in our blog post CRISPR 101: Validating your Genome Edit.
Publications
- Cong, L., & Zhang, F. (2015). Genome engineering using CRISPR-CAS9 system. Methods in Molecular Biology. 1239, 197–217. PMID: 25408407
- Hashimoto, M., & Takemoto, T. (2015). Electroporation enables the efficient mRNA delivery into the mouse zygotes and facilitates CRISPR/Cas9-based genome editing. Scientific Reports. 5, 11315. PMID: 26066060
- Zuris, J. A., Thompson, D. B., Shu, Y., Guilinger, J. P., Bessen, J. L., Hu, J. H., Maeder, M. L., Joung, J. K., Chen, Z., & Liu, D. R. (2015). Cationic lipid-mediated delivery of proteins enables efficient protein-based genome editing in vitro and in vivo. Nature Biotechnology. 33(1), 73–80. PMID: 25357182